Tideway Central – Junctions (2021)
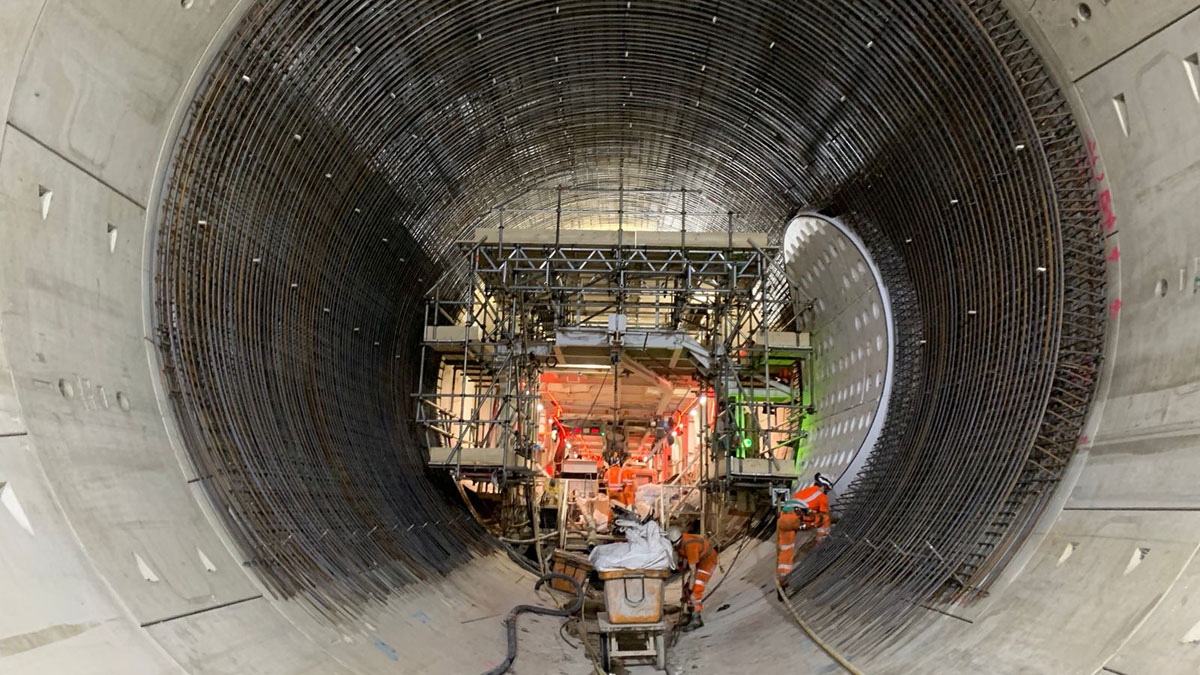
Fixing reinforcement for the main tunnel secondary lining at the junction location - Courtesy of FLO JV/Tideway
The Tideway Tunnel is a new 25km long combined sewage storage and transfer system that will help protect the ecology of the River Thames by tackling the problem of overflows from London’s ageing sewers. The Central Section consists of eight work sites each incorporating a drop shaft, as well as two tunnel drives. Of the shafts, six are ‘off-line’ which are connected to the main tunnel via connection tunnels, whilst the remaining two drop shafts are ‘on-line’ through which the main tunnel passes directly. This article focuses on the design of the tunnel linings for the junctions between the precast segmentally lined main tunnel and the sprayed concrete lined (SCL) connection tunnels. With the aid of advanced numerical analysis, a unique design solution was achieved which eliminated the need for additional temporary supports, whilst overcoming challenging ground conditions and extremes of loading.
Tunnel Junction Sites
This article focuses on the design of the junction of the primary linings between the connection tunnel and the main tunnel at the ‘off-line’ sites:
- Falconbrook Pumping Station (FALPS).
- Cremorne Wharf Depot (CREWD).
- Chelsea Embankment Foreshore (CHEEF).
- Heathwall Pumping Station (HEAPS).
- Albert Embankment Foreshore (ALBEF).
- Victoria Embankment Foreshore (VCTEF).
All the principal structures were constructed with both primary and secondary linings. The main tunnel primary lining at the junction location is formed from steel fibre reinforced and traditionally reinforced precast concrete segmental rings, whilst the connection tunnel primary lining is formed from steel fibre reinforced sprayed concrete lining (SCL). A key requirement of the design solution for the junctions was that there should be no programme link between the construction of the connection tunnels and the construction of the main tunnel.
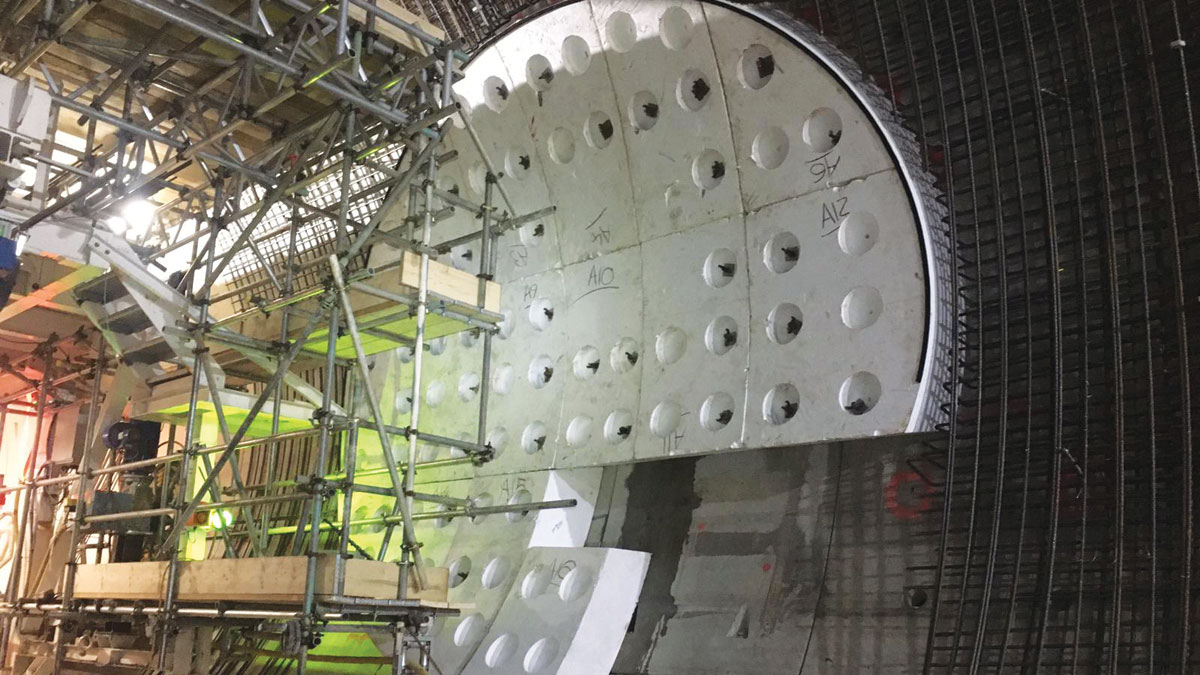
Reinforcement fixing for main tunnel secondary lining – Courtesy of FLO JV/Tideway
The connection tunnel breakthrough is to be made after the secondary lining in the main tunnel is cast. Therefore, there was the option to use the secondary lining in the main tunnel to support the segmental primary lining during breakthrough, giving the opportunity to optimise the temporary works. There are very limited case histories that use only external support without using opening sets. One such example is the Victoria Station Upgrade (VSU) project in which both longitudinal and diagonal steel props were installed at the extrados of the precast concrete segmental lined platform tunnel. In contrast, the only external support for the Tideway junctions is provided by the connection tunnel sprayed concrete lining itself. This is a unique and innovative solution which was developed in conjunction with the CAT III checker, Dr. Sauer & Partners.
Design challenges
There were a number of principal concerns with the design of the junctions for the project.
- Junction size: The ratio between the enlarged sections of the connection tunnels, and the main tunnel, are between 0.56 and 0.67. A typical rule of thumb for the design of tunnel junctions is that the ratio between the two tunnel diameters should not exceed 0.67.
- Junction geometry: All junctions are made at acute angles ranging between 60° and 70°, unlike conventional soft ground junctions which are commonly perpendicular. The acute connection results in a far more complex structural analysis than is the case for a perpendicular junction.
- Ground conditions: Only two of the junctions are located wholly within London Clay (where construction is more straightforward), whilst the remainder lie within mixed ground of varying complexity. This raised additional risks to be considered and mitigated during the design, particularly in relation to groundwater control. Broadly, the junctions can be categorised into two groups according to the ground conditions (upstream and downstream).
- Extremes of loading: There is a large variability in operational conditions which includes an empty system case, a maximum internal surge pressure case and a long-term future development load case. The internal surge case represents the maximum sewage water level that can be reached before the overflow provisions of the system become operational. This causes large tensile hoop forces in the combined tunnel lining system for which the concrete crack widths must be adequately controlled. The future development load case considers the potential for future development occurring above the tunnel which may impart additional unbalanced surcharge loading on the tunnel junction.
Design development and numerical analysis
The design of the junction is a complex problem that involves both non-linear soil-structure interaction and non-linear interactions between structural elements. The latter are due to the existence of radial and circumferential joints in the segmental lining. In line with current best design practice, the junction has been designed using a combination of modelling packages and hand calculations.
Complex soil-structure interaction was simulated using the geotechnical finite element software PLAXIS 3D. This allowed the impact of the staged construction of the SCL connection tunnel on the completed main tunnel to be modelled, resulting in more realistic ground pressures and ground stiffness at the junction. The software has advanced soil constitutive models enabling it to simulate the non-linear strain hardening behaviour of soil.
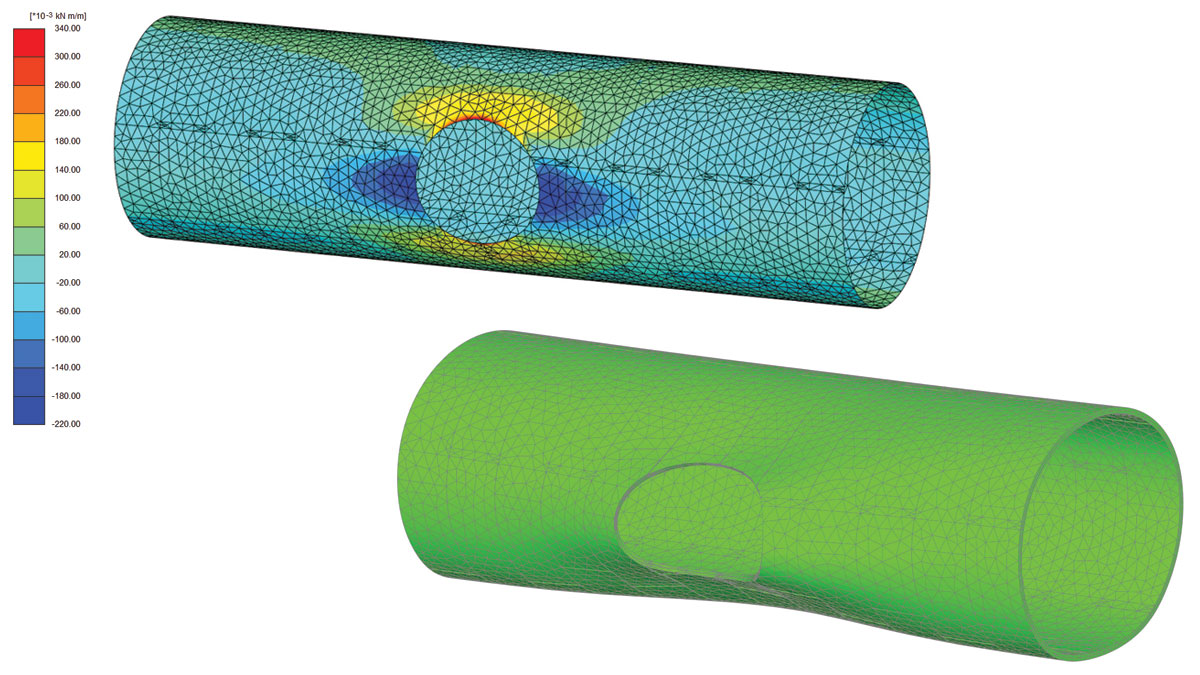
(top) PLAXIS 3D main tunnel primary lining bending moment in hoop direction for the long term permanent case (empty tunnel) and (bottom) PLAXIS 3D main tunnel primary lining deformed shape for the long term permanent case (empty tunnel) – Courtesy of AECOM
Simulation of main tunnel linings
The main tunnel primary lining is subject to unbalanced loading with resulting lateral displacement and bending moments during the construction of the junction. Accurate modelling of the primary lining in the earlier construction stages is important, as it is initially the only structural element providing resistance to the applied loads, until the secondary lining is installed (just before the main tunnel breakout).
The modelling approach adopted for the main tunnel primary lining consists of individual volume elements representing each 1.8m wide ring, with a low stiffness ‘dummy’ plate placed at the centreline of the elements in order to obtain structural forces. Volume elements were chosen as they allow contact forces to be generated between rings along the longitudinal direction of the tunnel.
The behaviour of the main tunnel is governed, in part, by the circumferential and radial joints between each precast segment of the primary lining. In order for differential movement to occur along the circumferential joints, frictional resistance between the concrete surfaces of each segmental ring must first be overcome. Only then is further shear resistance provided by the shear dowels.
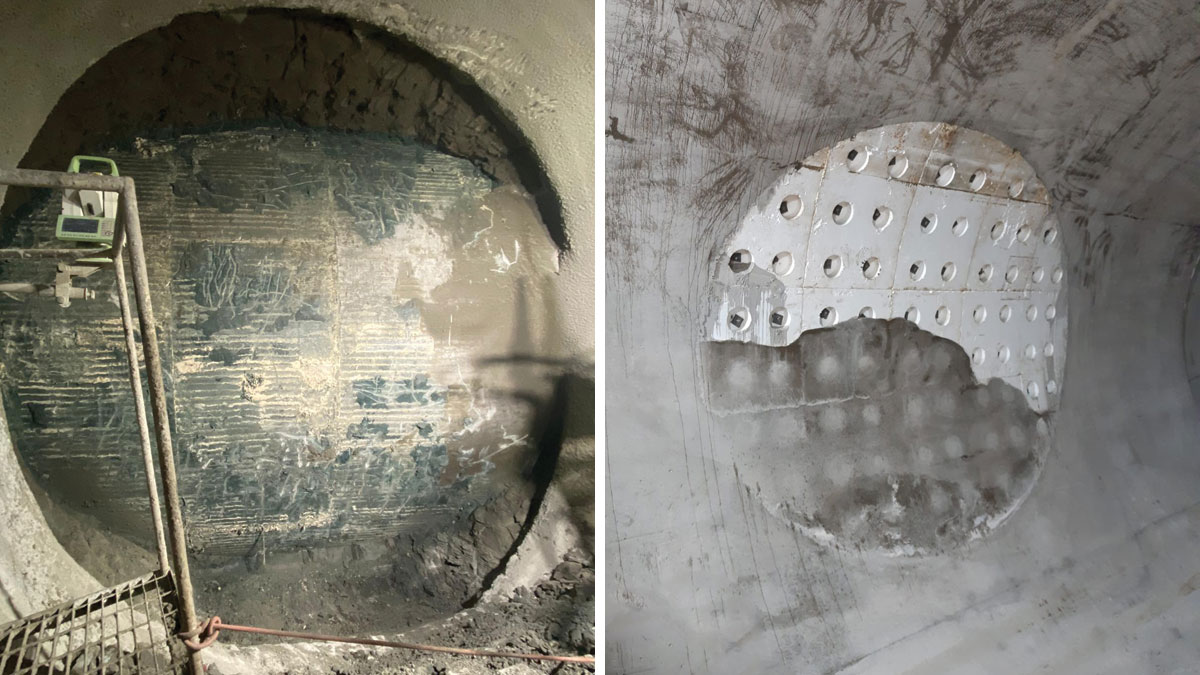
(left) Main tunnel primary lining rings exposed after connection tunnel excavation and (right) connection tunnel eye after casting main tunnel secondary lining – Courtesy of FLO JV/Tideway
Circumferential joints are modelled using frictional interfaces between the volume elements. These interface elements, with a cohesion of zero, allow each ring to move apart under tension, but generate contact forces in compression.
Radial joints between segments were accounted for by assigning a reduced flexural rigidity to the continuous volume that represents each ring, whilst maintaining the same axial rigidity, after Wood (1975).
This was preferable to the alternative of modelling individual radial joints using interface elements, which would have over-estimated the articulation of the segments by failing to account for the tensile stiffness contribution of the bolts between segments.
Simulation of connection tunnel
The numerical analysis considers the construction of the connection tunnel SCL primary lining in 1m advances towards the completed main tunnel, comprising unsupported excavation of each ring with the lining installation following behind.
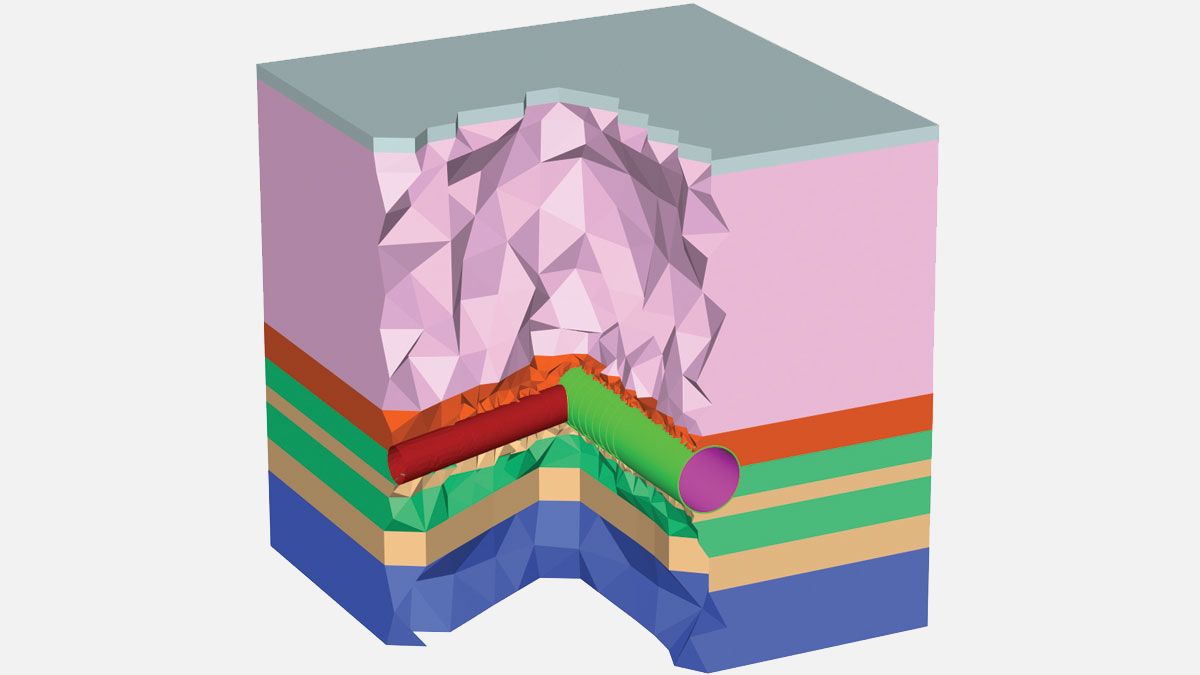
Cut-away view of the numerical model of the tunnel junction – Courtesy of AECOM
Main tunnel secondary lining geometry
In order to increase structural capacity at the junction location, the main tunnel secondary lining was given an increased thickness on the connection tunnel side of the junction, over a length of 12.6m centred on the junction.
Within this zone, the intrados of the secondary lining follows a half-ellipse profile, creating an increased thickness (450mm) at axis level which decreases back to 250mm at the crown and invert. The opposing half of the main tunnel secondary lining is subject to less severe loading and therefore adopts the same 250mm thickness as the rest of the tunnel.
The thickened region was sub-divided into a number of zones which allowed reinforcement layouts to be tailored to the forces occurring locally in each zone. The thickness at invert level is consistent with the rest of the tunnel and hence the required fall for the hydraulic performance of the tunnel is not compromised.
Thames Tideway Central Section: Junctions: Supply chain
- Project manager & principal designer: Tideway (Jacobs)
- Principal contractor & lead designer: FLO JV
- Main designer (permanent works): AECOM
- Main tunnel secondary lining formwork: TECOZAM
- SCL designer: COWI
- Durability consultant: OTB Concrete
- CAT III checker: Dr. Sauer & Partners
- Sub-consultants (temporary works): PERI
- Sub-consultants (temporary works): Hunnebeck
- Sub-consultants (temporary works): Laing O’Rourke
- Sub-consultants (temporary works): Robert West
- Sub-consultants (temporary works): Fairhurst
- Dewatering contractor: WJ Groundwater
- Main tunnel contractor: FLO JV
- Connection Tunnel SCL contractor: Joseph Gallagher Ltd
- Connection Tunnel SCL contractor: Barhale
- Connection Tunnel SCL contractor: FLO JV
- Instrumentation & monitoring contractor: FLO JV
- Sprayed concrete: CEMEX
- Main tunnel precast concrete segmental lining: Pacadar
- TBM: NFM Technologies
- Secondary lining formwork: DOKA
- Secondary lining formwork: Specialist Formwork
- Cast in situ concrete: Hanson
- Steel: Express Reinforcement
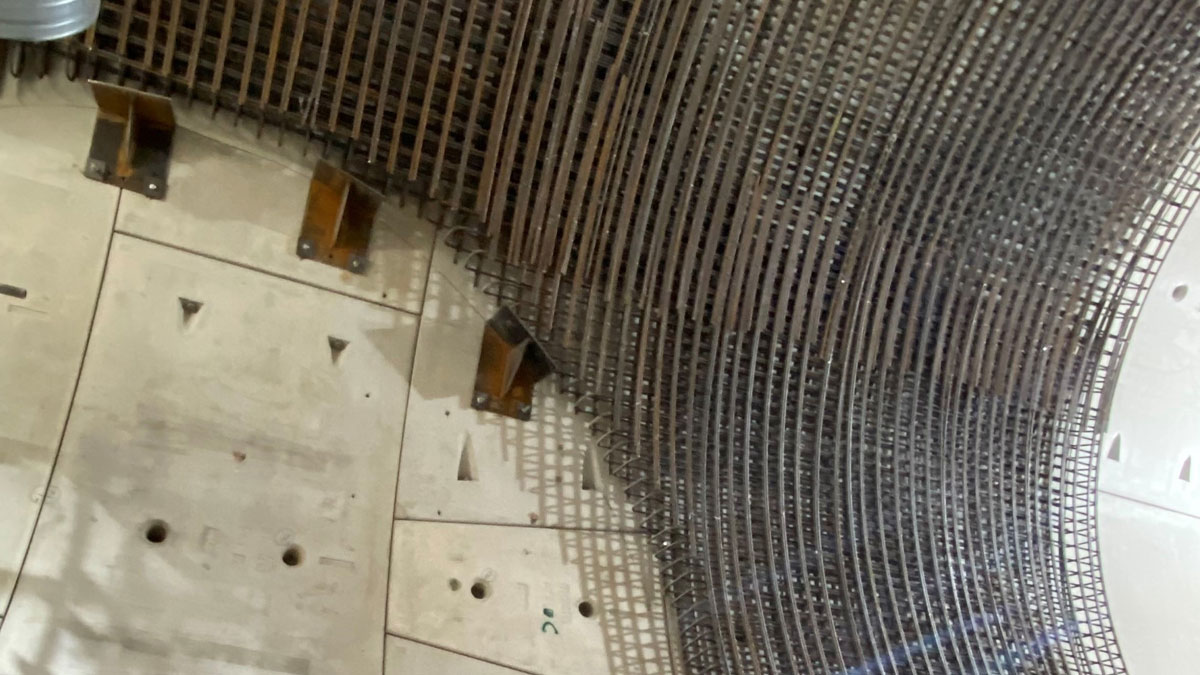
Reinforcement fixing for the main tunnel secondary lining around the connection tunnel eye – Courtesy of FLO JV/Tideway
Mitigation of geotechnical risks
The existence of granular water bearing materials in the connection tunnel face at the ‘downstream’ junctions would have led to local instability of the excavation if not treated.
Therefore, a set of ‘toolbox’ methods was developed to accommodate issues that may arise. These included:
- Pre-drilled grouted spiles to be installed where the excavation is not stabilised by an initial 75mm sprayed concrete lining layer.
- Reducing excavation round lengths to less than 1.0m.
- Temporary 75mm SCL sealing layer and water relief pipes for depressurisation in the event that water flow is observed on the excavation face.
- Dewatering of granular material (where it exists below invert level) to control invert heave.
Face inspection and logging was carried out to identify shear zones and areas of low undrained shear strength where pocket excavation may be necessary.
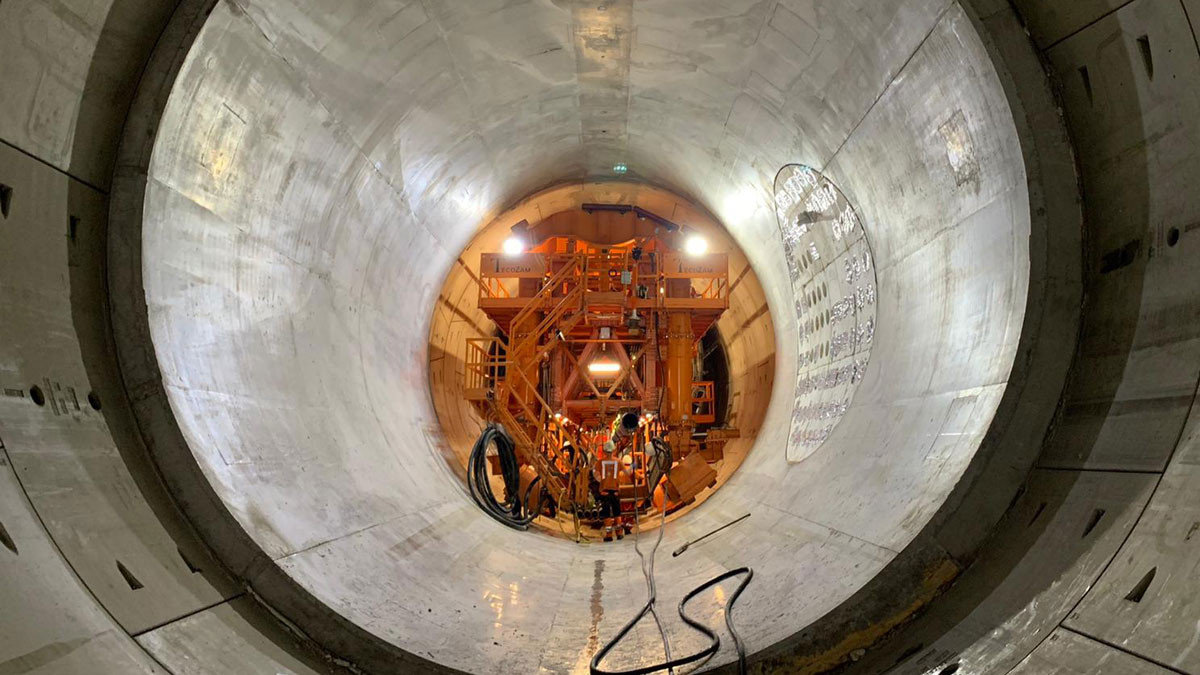
Main tunnel secondary lining half ellipse profile – Courtesy of FLO JV/Tideway
Conclusions
A construction approach that utilises the main tunnel secondary lining to provide support to the junction opening, without pre-installation of any internal props was found to be feasible and was implemented for the junction sites.
At the time of writing (June 2021), the break-out of the main tunnel primary lining is underway at the junction sites. This will be followed by casting of the secondary lining in the connection tunnel, which will complete the junction construction.